2030 Doomsday Scenario: The Great Nuclear Collapse
A timeline of the end game for human civilization
Humanity has constructed a doomsday Deadman switch that threatens civilization. Climate destruction will make it increasingly difficult to avoid the looming global nuclear catastrophe we've created.
Here's how our future might unravel:
Late 2020s: Climate Red Alert and Infrastructure Strain
By the late 2020s, Earth’s climate is in unprecedented turmoil. Global average temperatures are consistently 1.5 °C above pre-industrial levels. Each year brings record-breaking heatwaves, “freak” floods, and droughts that batter infrastructure. Coastal cities flood more frequently, roads buckle in extreme heat, and power grids strain under surging demand for cooling.
This cascade of climate disasters sets the stage for a systemic collapse: as societies grapple with runaway warming, the resilience of critical infrastructure (power, water, transit) erodes.
Energy systems enter a crisis even before 2030. Nuclear power, which in 2025 still provided about 9% of the world’s electricity from ~440 reactors, becomes increasingly unreliable. Many nuclear plants struggle with climate stresses: cooling water sources heat up in summer, forcing reactors to reduce output or shut down to avoid unsafe temperatures. For example, a 2028 European heatwave pushes river and sea temperatures above 25 °C, triggering emergency shutdowns at multiple reactors that cannot be cooled effectively.
At the same time, stronger storms and floods threaten reactor safety. Dozens of reactors worldwide are unprepared for extreme flooding, meaning a dam failure or storm surge could lead to a Fukushima-scale accident. Worrisome reports emerge of power plants in floodplains and coasts where defenses are overtopped by rising seas and torrential rains.
By 2029, global carbon output remains high, and natural feedback loops are kicking in. In the Arctic, permafrost thaws and releases methane creating a vicious warming cycle where initial warming triggers more emissions, leading to even more warming. Scientists caution that a tipping point is near, beyond which climate change becomes self-perpetuating (a true “runaway” scenario).
Society approaches 2030 in a precarious state: aware of looming catastrophe yet unprepared for its speed. The stage is set for the coming collapse, with power grids and nuclear facilities - the backbone of the industrial world - already under severe strain.
Early 2030s: Blackouts and the First Reactor Crises
2030 marks the breaking point.
A confluence of climate catastrophes collapses power grids across multiple continents. A severe global heatwave in the summer of 2030 brings record electricity demand while many power plants (nuclear and coal alike) are derated or offline due to overheating coolant water.
Then powerful Category 5 storms strike in succession: one hurricane inundates the U.S. Eastern seaboard, while an unprecedented typhoon swamps Southeast Asia. These disasters knock out transmission lines and flood key substations, leading to prolonged blackouts in dozens of major cities. Emergency systems are overwhelmed. With communications down and transportation paralyzed, manpower shortages become acute - many operators and engineers cannot reach their stations.
Nuclear power plants are among the first to feel the emergency. Grid failure triggers automatic reactor SCRAMs (rapid shutdowns) at plants from Florida to France. Control rods halt the fission reactions, but decay heat in reactor cores still needs cooling for days to prevent meltdown.
Normally, backup diesel generators would power the cooling pumps, but the scale of the blackout means diesel resupply is uncertain and some generators fail in flooded facilities. In a grim reflection of 2011’s Fukushima disaster, several coastal reactors lose all power as storm surges drown their backup generators.
Within hours to days, the first meltdowns occur.
In 2031, a reactor in South Asia becomes a flashpoint: its cooling pumps falter after the grid collapse, leading the core to overheat. The reactor’s heart melts through containment in a matter of days, releasing a plume of radioactive steam and debris.
Nearby, an even greater danger unfolds: the plant’s spent fuel pool, packed with years of highly radioactive spent rods, boils dry without cooling. Exposed to air, the zirconium cladding of the fuel ignites, triggering a fire that belches long-lived radioisotopes directly into the atmosphere. This nightmare scenario - once narrowly avoided at Fukushima by heroic ad-hoc measures - now plays out in full.
Local and regional consequences are immediate and harrowing. Authorities, already struggling with disaster response, hastily order mass evacuations around stricken plants. In the South Asia incident, a radius of 30 km is declared a no-go zone as radiation levels spike. Over one million people are displaced in this region alone, fleeing what swiftly becomes a nuclear dead zone. Many receive significant radiation doses during the chaotic evacuation, trapped by traffic jams under drifting fallout.
Comparisons are made to Chernobyl’s 1986 evacuation – there, 130,000 people were permanently resettled and a 1,000-square-mile exclusion zone established – but the 2031 event affects an even larger population in a densely settled area.
Nearby countries track the radioactive cloud as it crosses borders. Within days, radioactive iodine and cesium are detected in cities hundreds of kilometers downwind. Governments distribute iodine tablets to help block uptake of radioactive iodine in thyroid glands, recalling measures taken after Chernobyl and Fukushima. Farmers in downwind regions watch in despair as cesium-137 contaminates soil and crops, knowing from past accidents that those lands may be unsafe for farming for decades. (After Chernobyl, for instance, radio-cesium lingering in soils kept pastures in parts of Europe under restriction for over 20 years.)
Globally, these first reactor crises send a chilling signal. Airborne radiation from the fires and vented steam reaches the upper atmosphere and begins circling the planet. Within weeks, trace amounts of cesium-137 and strontium-90 are found in faraway monitoring stations.
While the initial fallout poses the greatest danger locally, the global dispersion of radionuclides raises alarms. Public health experts warn that even low-dose fallout on crops could, when multiplied across the world, elevate cancer risks and contaminate food supplies. International markets are rocked as nations ban produce and grain imports from entire regions. The economic shock compounds the physical destruction: already destabilized by climate disasters, the global supply chain further fractures under fear of radiation in goods.
Perhaps most critical for what comes next, these early accidents erode the capacity to respond to future crises. Emergency workers who heroically battled the first meltdowns (hosing overheating reactors, attempting improvised cooling) have suffered radiation exposure or exhaustion. Large swaths of power grid remain offline, making rolling blackouts the new normal even in areas not directly hit by climate events. This energy shortage slows recovery efforts and undermines the cooling and monitoring systems at other nuclear sites. By 2032, the world faces a stark reality: roughly 10% of nuclear reactors worldwide are in some stage of crisis - either already melted down, or scrammed and struggling to keep their hot cores and spent fuel safe. What was once unthinkable now seems inevitable.
Mid-2030s: Cascading Meltdowns Across the World
As 2035 approaches, the situation spirals into a cascade of nuclear calamities. Ongoing climate chaos keeps hammering human systems. Year after year, megastorms, wildfires, and heatwaves pummel regions before they can recover. The compounded infrastructure damage means many areas have only intermittent electricity and scarce supplies.
In this environment, about half of the world’s nuclear reactors are effectively left unattended or unserviceable – some due to direct disaster impacts, others because manpower and resources have collapsed in the region. Governments in relatively stable areas attempt to initiate orderly shutdowns of reactors as a preventative measure, but even a shut reactor needs years of active cooling and oversight. In many cases, those best efforts falter.
By 2033–2035, a wave of reactor meltdowns unfolds on nearly every continent.
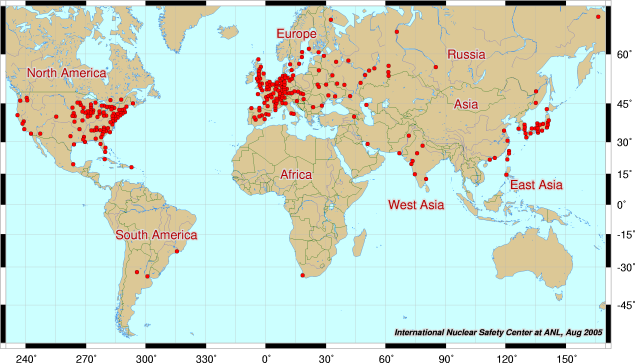
The numbers are staggering. What started with a few isolated accidents in 2030–32 explodes into dozens of sites in crisis. Older nuclear stations prove especially vulnerable: lacking passive cooling features, they succumb quickly when grid power and backups fail. Newer reactors touted as “meltdown-proof” also face unforeseen challenges – coolant reservoir tanks run dry when maintenance crews vanish, or hydrogen explosions (like those that blew apart Fukushima’s reactor buildings) occur due to unvented pressure.
Spent fuel pool fires add to the nightmare at many sites; analysts later estimate that these pool fires released even more radiation than the reactor core meltdowns in several cases, since pools often contained decades of fuel assemblies (holding up to 10× the long-lived radioactivity of a reactor core in each pool).
Each collapsing plant creates its own radiation footprint. By the mid-2030s, a patchwork of radioactive exclusion zones scars the Northern Hemisphere. In Eurasia, multiple zones – from Western Europe through Russia, South Asia, and East Asia – dot the map where reactors have failed. Some of these zones begin to overlap, forming a virtually continuous swath of contaminated land in parts of Europe and Asia.
In Western Europe, for example, meltdowns at two French reactors and one German reactor in 2034 force evacuations that cover large parts of the Rhine valley. Later, a catastrophe at Ukraine’s Zaporizhzhia plant (already endangered for years prior) adds to the chain, rendering areas along the Dnieper River highly radioactive once again.
North America is not spared: a meltdown at an aging Midwest U.S. plant sends radiation across several states, and Canada’s Ontario reactors - shut down due to power loss - suffer a fuel pool fire that spreads contamination through the Great Lakes region.
In total, roughly 50% of the world’s 400+ reactors are now either destroyed or abandoned. Humanity suddenly finds itself living with hundreds of Chernobyl-sized disasters at once.
Local and regional consequences reach an apocalyptic scale. Hundreds of millions of people become actual or potential refugees from high-radiation areas. Major cities near failed plants are emptied: by 2035, regions like the French Riviera, the North China Plain, and the U.S. eastern seaboard have pockets that resemble Pripyat – ghost cities left to wild animals.
The contamination of land and water is immense. Isotopes like cesium-137 and strontium-90 settle into agricultural soils. Just as Chernobyl’s fallout once contaminated 200,000+ square kilometers of Europe to some degree, the 2030s meltdowns contaminate vast expanses of the globe. Agricultural experts estimate that a significant fraction of the world’s breadbaskets are now tainted by radioactive fallout.
For example, the Punjab region and the American Midwest both see cesium levels in soil far above safe farming limits, threatening global grain supplies. In many countries, the choice is stark: eat potentially contaminated food or starve.
Livestock that graze on fallout-blanketed pastures accumulate radionuclides in their meat and milk, as British sheep did for decades after Chernobyl. Governments impose strict bans on food exports from these zones, and global food prices skyrocket. Famine looms for countries that relied on imports from now-irradiated farmlands.
Beyond human habitations, ecosystems suffer radiological damage layered on top of climate stress. Forests downwind of reactor accidents turn brown and silent as foliage and wildlife absorb heavy doses of radiation. In some intensely contaminated zones, an eerie calm prevails – reminiscent of how the core area around Chernobyl became an accidental wildlife refuge, but one where many organisms die young or show mutations.
Initially, high radiation kills or stunts many plants and animals. Forests die and animal populations plummet. Over the later 2030s, some wildlife returns to abandoned zones, benefiting from the lack of humans. However, in areas of very high contamination, biodiversity remains lower and animals show signs of chronic radiation exposure.
The web of life is poisoned: radioactive cesium and strontium work their way up food chains, affecting predators and prey alike. Combined with the ongoing climate upheavals (heat stress, wildfires, habitat shifts), the added burden of radiation pushes many species to extinction in contaminated regions. Aquatic ecosystems are also hit – radioactive runoff flows into rivers and seas, causing fish kills and long-term mutations in fish reproductive cycles.
The global consequences of this mid-2030s nuclear cascade are profound. Atmospheric circulation transports radioactive pollution around the world. By 2035–2036, background radiation levels have risen noticeably above 2020s norms in both hemispheres. Radioactive particles from multiple meltdowns are detected in the Arctic and even the Antarctic, having been carried by air currents. Although concentrations far from accident sites are low, no corner of the planet is truly untouched.
In the Northern Hemisphere, intermittent waves of fallout descend whenever rain clouds scavenge particles from the upper atmosphere – a phenomenon similar to the fallout patterns observed after nuclear weapons tests and Chernobyl, but now sustained by ongoing reactor fires and spent-fuel blazes. Public health experts warn that long-term cancer rates will climb worldwide; every additional becquerel in our food and water increases risks.
By the late 2030s, the world’s socio-economic order has largely disintegrated. The combination of climate catastrophe and radioactive contamination fractures the globalized economy. International travel is nearly nonexistent both because of infrastructure breakdown and fear of radiation exposure on long journeys. Trade in food and goods has devolved into ad-hoc local barter, since centralized distribution is impossible under constant disaster.
Regions that remain habitable form “safe zones” - relatively less contaminated and with tolerable climate - mostly in the far southern hemisphere and a few remote northern areas. For instance, parts of New Zealand, Patagonia, and Siberia (far from any meltdown sites and somewhat buffered by distance) become refuges for those able to relocate. Even so, these areas face their own challenges from extreme weather and inflows of refugees.
Humanity’s population shrinks precipitously due to famine, conflict, and radiation-related illness. What was roughly 8 billion people in 2020 falls by at least hundreds of millions (edit: more likely billions) by 2040. Those losses stem not only from immediate disaster casualties but also from secondary effects: hunger, lack of medical care, and weakened immune systems in a ravaged environment.
2040s: The Toxic Legacy Settles In
By the 2040s, the frantic pace of new catastrophes slows somewhat – not because the crises are solved, but because so much has already collapsed. Most of the vulnerable nuclear reactors have already broken down by this point or were pre-emptively shut. The ones that survived the 2030s are primarily in regions that remained functional enough to manage a safe cold shutdown or have newer designs with passive cooling. However, the world now faces the long aftermath of what has happened. The 2040s are a bleak decade of enduring fallout (literal and figurative), where humanity grapples with the toxic legacy of hundreds of reactor failures amid a climate that remains hostile.
One grim reality sets in: the radioactive contamination is far from a short-term problem. Many of the isotopes released have half-lives measured in decades or longer, meaning the radiation will persist for generations. For example, cesium-137 (half-life ~30 years) and strontium-90 (half-life ~29 years) remain abundant in the soils of meltdown zones and downwind regions.
These isotopes mimic vital nutrients (cesium behaves like potassium, strontium like calcium), so they continuously cycle through plants, animals, and water. Crops grown in contaminated soil uptake cesium; grazing animals concentrate it in their flesh; humans who consume those foods further concentrate it in their bodies. In the 2040s, scientists document how radioactivity has infiltrated the global food chain. Traces of cesium-137 show up in grain and milk even in “safe” zones, due to minute fallout that has spread worldwide.
In harder-hit areas, food contamination remains a severe obstacle to resuming agriculture – even when farmers attempt to cultivate, their produce often exceeds safety limits imposed in the old world. Consequently, hunger continues to stalk populations: arable land might be available, but not all of it can be used without slowly poisoning those who eat from it.
Another challenge is the management of radioactive waste and materials. The reactor meltdowns and fires have dispersed a lot of the radioactive inventory into the environment, but significant amounts still reside in the wreckage of power plants.
Spent fuel rods that did not burn sit in cracked pools or dry casks at sites now too hazardous for people to approach. The reactors themselves hold tons of uranium and plutonium in their ruined cores. In the 2040s, these wreckage sites are largely uncontained.
Unlike Chernobyl, where a concrete “sarcophagus” was built over the destroyed reactor, many 2030s accident sites have been simply abandoned mid-disaster. Some have rubble or sand piled by drones or remote machines to try to smother fires, but no comprehensive containment. This means groundwater leaching becomes a major concern. Rain percolating through the wrecked reactors carries radioactive contaminants into aquifers and rivers.
For communities downstream (if any remain), water sources are compromised. In coastal plants, continued leakage of radiation into the ocean is observed. By 2045, marine biologists report increased contamination in sea life far from any direct fallout, indicating that ocean currents have spread the pollutants. Strontium-90, for instance, known to accumulate in fish bones, is found in fish thousands of kilometers from any reactor site. The Pacific Ocean, already contaminated by the Fukushima incident in 2011, now receives orders of magnitude more radionuclides from multiple Pacific Rim reactor failures.
Ocean fisheries, already stressed by climate-driven acidification and overfishing, are now additionally burdened by radioactive pollution – many fishing zones are closed due to cesium levels, pushing more coastal communities into protein scarcity.
The climate crisis continues unabated in the 2040s, though its character has changed. With industrial civilization greatly diminished by mid-decade, greenhouse gas emissions from human sources have plummeted. Oil consumption is a fraction of what it was, and many coal plants are offline (some destroyed, some simply without supply lines). This initially gives a glimmer of hope that anthropogenic warming might slow.
Indeed, by the late 2040s some climatologists note a slight stabilization in CO₂ levels. However, the damage is already done in terms of triggering feedback loops. Warming continues due to inertia and feedback emissions (like methane from permafrost). By 2040 the world breached +2 °C (edit: likely more like 3) warming, and by 2050 it may be heading toward 2.5 °C (edit: quite possibly approaching 4.5) despite the collapse in human emissions.
The ongoing extreme weather further complicates the radioactive legacy. For example, wildfires in contaminated forests have become a recurring nightmare. Each summer in the 2040s, large wildfires ignite in areas with dry, hot conditions – some of those areas include the evacuated zones dense with dead trees and dry brush (around former reactor sites). When these fires rage through radioactive forests, they loft radionuclide-laden smoke into the sky.
In 2043, a massive fire in the abandoned parts of Eastern Europe (fueled by a drought and heatwave) burns hundreds of thousands of acres, re-mobilizing cesium and plutonium deposited in the soil. Soot and ash carrying these particles travel far; monitors as far away as northern Scandinavia register spikes in airborne radiation. What was effectively “locked” in the soil is thus released anew by fire – a horrific feedback where climate-induced fire boosts the spread of nuclear contaminants.
Similarly, intense storms cause flooding and dust storms that redistribute radioactive sediments. Rivers that flow through meltdown zones periodically flood and deposit radioactive silt onto downstream plains. The environmental contamination, therefore, is not a static situation; it worsens in pulses whenever climate disasters strike the polluted zones, creating secondary fallout events throughout the 2040s.
Human society in this decade adapts in grudging, hardscrabble ways. In relatively uncontaminated regions, people develop new habits to minimize radiation exposure. For example, rooftop farming and hydroponics indoors become crucial to grow food in controlled environments, to avoid contaminated soil. Water is filtered through improvised means (layers of charcoals and resins to trap radioactive isotopes). People often wear personal dosimeters and masks when venturing outside, especially on windy days that could carry dust. The specter of radiation sickness and cancer is a constant part of life.
Medical knowledge from past nuclear accidents is applied where possible. For instance, Prussian blue pills (which bind radioactive cesium in the digestive tract) are prized treatments to reduce cesium uptake; potassium iodide pills are stocked to pre-dose the thyroid in case of new radioactive iodine releases. However, these medications are in short supply as global production capacity and supply chain infrastructure is decimated.
Despite these measures, the health toll is severe. Cases of cancers (thyroid, leukemias, solid tumors) skyrocket, and with healthcare systems devastated, many go untreated.
There is also a rise in birth defects in regions that were exposed to higher radiation during the 2030s – a tragic echo of what was observed in some areas after Chernobyl, now magnified by the wider scale. Mental health is another casualty: whole generations grow up under the dual shadow of climate apocalypse and invisible radiation hazard, leading to widespread psychological trauma and “eco-radiation anxiety.”
By the end of the 2040s, some stabilization occurs in the sense that no new major nuclear disasters are unfolding (simply because so few reactors remain operational or intact). What remains of organized governments and international institutions focus on containment and mitigation. There are projects, for instance, to entomb certain high-risk reactor sites in concrete (as was done with Chernobyl) now that radiation levels around them have decayed enough to allow heavy machinery to approach for short periods. One such international effort in 2048 finally encases the remains of a major U.S. reactor that melted down 15 years prior, using robotic builders to minimize human exposure. These efforts are slow and cover only the worst offenders, but they at least aim to prevent further leakage.
2050s and Beyond: A Transformed and Radioactive World
Earth is a fundamentally altered planet. Human civilization has been gutted; what remains is a patchwork of survivor communities and a few stable enclaves attempting to rebuild amid the ruins. The climate is hotter (approaching +2.5 °C), seas are higher, and seasons are unreliable. On top of this, the planet’s surface carries the wounds of the nuclear collapse. Even as some dangers gradually subside with time, others will persist for centuries.
Radioactive decay has slightly improved conditions in the decades since the meltdowns. By 2060, it will have been ~25–30 years since the peak of the disaster. Isotopes like Iodine-131 (which caused acute thyroid exposures in 2030s) are long gone – with an 8-day half-life, they decayed away within months of release. The most intense short-term radiation from the accidents (which came from these short-lived fission products) has thus faded. Even some medium-lived isotopes like cesium-137 and strontium-90 have seen about one half-life pass. Areas that were extremely contaminated by cesium in 2035 might register roughly half the cesium levels by 2065, simply due to radioactive decay (not counting redistribution). This means that radiation levels in some exclusion zones are lower in 2060 than they were in 2040, potentially allowing limited access with protective gear.
In a few zones on the periphery of disasters, radiation has decayed enough that authorities consider letting people return with precautions (much like parts of the Fukushima exclusion zone were gradually reopened after a decade). Wildlife begins to reclaim many regions more fully as human absence continues; in moderately contaminated areas, animals have multiplied (albeit some with shorter lifespans or health effects). The paradox seen in Chernobyl’s exclusion zone – where wildlife thrives despite radiation because human pressures are removed – is now playing out on a larger scale. Some scientists in the 2050s cautiously talk of certain abandoned areas becoming de facto wildlife reserves, albeit radioactive ones.
However, other hazards will essentially be permanent on human timescales. One is plutonium. Many reactor explosions and fires spread particles of plutonium-239, an alpha-emitting isotope with a half-life of 24,000 years, into the environment. These particles are extremely dangerous if inhaled or ingested, as they can lodge in lungs or bones and irradiate tissue for a lifetime. Plutonium is heavy and tends to deposit near accident sites, but the fires and smoke did carry some of it regionally. This means certain hotspots (within, say, a few kilometers of the worst meltdowns) will remain lethally radioactive essentially forever as far as human planning is concerned.
Even after cesium decays, these areas will be unsafe to inhabit without serious cleanup (removal of topsoil, etc.). Another enduring issue is the spent fuel and waste that remain. By 2070, the fuel assemblies that did not burn up in fires have cooled radiologically (their short-lived fission products gone), but they are still highly radioactive and contain long-lived isotopes. Ideally, they would be secured in geologic repositories to isolate them from the biosphere. But with the collapse of industrial capacity, most of this waste is simply sitting wherever it was last stored. Some is in dry cask containers that can last a few decades. By the 2070s those casks may be deteriorating, potentially releasing their contents if not maintained. Thus, the world faces a slow seepage of radionuclides for centuries.
The habitability of the planet is dramatically reduced compared to pre-2030. Large regions are effectively off-limits due to radiation – especially parts of mid-latitude North America, Europe, and Asia where population was once highest. The tropics, meanwhile, suffer extreme heat and humidity that push human heat tolerance to the limit (some equatorial zones regularly see wet-bulb temperatures above 35 °C, unsurvivable without A/C).
The “safe zones” by the 2050s are those rare places with a combination of tolerable climate and minimal fallout. These tend to be in the southern hemisphere or isolated islands. Portions of South America (southern cone) and Africa (extreme south or highlands in East Africa) see clusters of survivors who have organized small agrarian societies, carefully selecting crops and livestock that can grow in changed conditions and relatively uncontaminated soils. Australia and New Zealand, which had no nuclear plants of their own and were distant from most fallout, become crucial harborages of technological memory – although Australia’s interior is severely hit by heat and drought, its southern coasts remain livable. Antarctica and the Arctic islands, free of radiation but harsh in climate, see some interest as refuges (some communities attempt to live in domed biomes on the Antarctic Peninsula, leveraging the cooler climate and abundant marine life, despite the logistical difficulties).
The collapse of industrial emissions has a small silver lining for climate by 2070: atmospheric CO₂ has finally plateaued, possibly even dipped slightly as the oceans and regrowing forests draw down carbon. But this comes at the cost of global societal collapse and mass mortality. In essence, the Earth system reset itself in part by a brutal reduction of human impact, while locking in a radioactive legacy. The climate remains warmer and more volatile than the Holocene average, but without continuous fossil fuel burning it may avoid worst-case 22nd-century projections. Nonetheless, sea levels by 2070 are higher (many coastal former cities are now tidal marshes littered with ruins), and superstorms still occur (though fewer targets remain to damage).
The surviving humans have adapted to a nomadic and subsistence lifestyle in many places, always mindful of avoiding radiation hotspots identified by their Geiger counters. The world population is a fraction of what it was, industrial civilization is dead alongside billions of humans, and those who remain are scattered and isolated.